Current Research
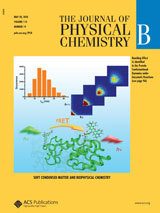
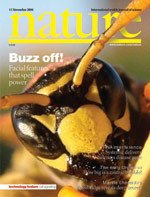
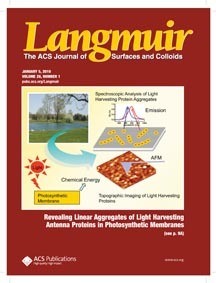
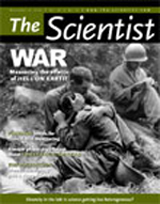
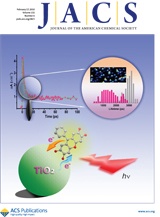
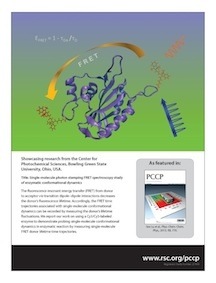
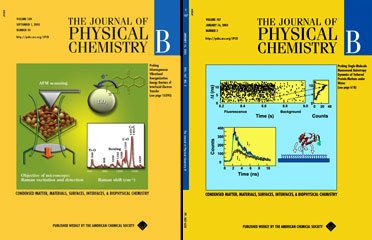
Current Research Scope
Our research is focused on the use of single molecule techniques to understand molecular dynamic processes and the effects of the local environment on these processes. We have been developing and applying time-resolved, nanoscale site-specific, single molecule methods that are an effective alternative to conventional methods, providing information under conditions most applicable to the natural processes underlying the area of research interest. Single-molecule approaches are useful and unique in studying heterogeneous and complex systems because the inhomogeneity can be identified and/or removed by studying one molecule at a time. Single molecules and molecular complexes can be observed as they traverse a wide range of energy states in real-time and the effect of this ever changing "system configuration" on chemical/biological reactions and other dynamical processes can be mapped.
Our current research work has been focused on (1) conformational dynamics and reaction in proteins and protein complexes under physiological conditions, and our long-term goal is to study single-molecule protein conformational dynamics and reactions in living cells; and (2) inhomogeneous interfacial chemical and biological reaction dynamics in solar energy conversion, bioremediation, and environmental systems, focusing on fundamental understanding of the controlling physical and chemical properties, such as, Franck-Condon coupling and barrier, vibrational and solvent relaxation energetics, molecular distributions, redox states identification, and molecular motions.
Although enzymatic reactions are traditionally studied at the ensemble level, the inhomogeneities of the reaction rate, the correlated enzyme conformational motions, and the non-synchronized nature of these reactions make it extremely difficult to obtain stepwise mechanistic and dynamic information from such studies. Presumably, the protein conformational motions at the active site of the enzyme are most critically responsible for the inhomogeneities in the enzymatic reactions. It will be critical in understanding the mechanisms and dynamics of intrinsic inhomogeneous and non-synchronizable enzymatic reactions to obtain real-time observations showing the correlation of single-molecule enzymatic reactions with unambiguously assigned protein conformational motions. The long-term goal of this project is to extend our molecule-level understanding of protein conformational fluctuation and relaxation dynamics and their impact on enzymatic reaction dynamics. Non-Arrhenius and non-Markovian dynamics, historesis, cooperativity, and memory effects of enzymatic reactions are widely observed but are insufficiently understood due to the nature of the inhomogeneity in an ensemble-averaged measurement. Applying single-molecule spectroscopy, we were able to probe protein conformational motions of a single enzyme (T4 lysozyme, an 18.6-kDa, single-subunit, and 164-amino acid enzyme comprising two domains connected by an α-helix) during hydrolysis of the polysaccharide walls of Escherichia coli B cells. By attaching a donor-acceptor (tetramethylrhodamine-Texas Red) pair of dye molecules site-specifically to non-interfering sites on the enzyme, we measured the dynamics of the hinge-bending motions of the enzyme under enzymatic turnovers by monitoring the donor-acceptor emission intensity changes due to single-pair fluorescence resonance energy transfer (spFRET). The overall enzymatic reaction rate constants were found to vary widely from molecule to molecule under the same physiological condition. The enzyme’s searching for reactive sites in the substrate was found to account for most of this inhomogeneity. By applying a molecular dynamics (MD) simulation and a random-walk model to analyze the enzyme-substrate complex formation dynamics, we have revealed multiple intermediate conformational states in the chemical reaction process. This approach provides information on the microscopic conformational change mean drifting velocity, diffusion coefficient, friction coefficient, energy consumed by friction along the reaction coordinate, and the energy landscape. This information cannot be obtained by an ensemble-averaged experiment, but is obtainable by a single-molecule approach. Our combined approach achieved the simultaneous measurement of enzyme conformational motion and enzymatic turnovers and demonstrates the potential of single-molecule studies in understanding non-synchronizable, multi-step reaction dynamics and mechanisms, eventually, in living cells.
Cell signaling is at the core of most biological functions and often involves dynamic interactions among proteins. Protein-protein interactions induce conformational changes that initiate chain reactions, which in turn lead to cellular responses. A comprehensive understanding of such protein interactions is crucial to understanding the regulatory mechanisms controlling cellular functions. To study protein interactions in cell signaling, ensemble measurements, which yield information only on averaged properties, are inadequate. Intrinsically, the crucial early events of cell signaling often involve only a few molecules and then are magnified along the signaling pathways. Moreover, for intrinsically heterogeneous systems such as protein complexes, protein interaction dynamics contain static and dynamic disorders as a result of spatial and temporal inhomogeneities. Stochastic protein-protein interactions prevent such characterizations when many molecules are measured simultaneously. Single-molecule spectroscopy is crucial to obtaining such information because it is capable of characterizing biomolecular processes that are inhomogeneous and non-synchronizable. We have conducted a single-molecule study of protein-protein interaction dynamics in an intracellular signaling protein complex, Cdc42/WASP, employing a novel CBD biosensor, which is a dye-labeled WASP fragment that binds only the GTP-activated Cdc42, we were able to probe hydrophobic interactions associated with Cdc42/WASP recognition. Single-molecule fluorescence measurements indicated static and dynamic disorders in this protein-protein interaction system, and our study characterized the dynamic and inhomogeneous nature of molecular recognition within the Cdc42/WASP signaling complex. A coupled-two channel fluctuation Markovian model was proposed for the fluctuation dynamics, and the molecular structure and solvent-accessible surface of the protein-protein interaction complex were explored by MD simulations. The Cdc42-CBD complexes showed conformational fluctuations between bound and loosely bound states while the overall complex was still associated. The distribution of the fluctuation rates was highly inhomogeneous, with variations among individual complexes of two orders of magnitude under the same conditions. The results suggest highly dynamic rather than static protein-protein interactions in this cell signaling system. Such dynamic and fluctuating protein-protein interactions may be important for inducing and regulating downstream signaling events. By establishing a molecular imaging system with adequate spatial and temporal resolution and combining single-molecule experimental and computational approaches, we expect to expand our studies to other important biomolecular complexes under physiological conditions and eventually to living cells.
Complex conformational changes influence and regulate the dynamics of ion channels in living cells. Such conformational changes are stochastic and often inhomogeneous, which makes it extremely difficult, if not impossible, to characterize them by ensemble-averaged experiments or by single-channel recordings of the electric current that report the open-closed events but do not specifically probe the associated conformational changes. We have conducted studies on ion channel conformational changes using a new approach, patch-clamp fluorescence microscopy (PCFM), which simultaneously combines single-molecule fluorescence spectroscopy and single-channel current recordings to probe the open-closed transitions and the conformational dynamics of individual ion channels. We demonstrated PCFM by measuring gramicidin ion channel conformational changes in a lipid bilayer formed at a patch-clamp micropipette tip under a buffer solution. By measuring single-pair fluorescence resonance energy transfer (spFRET) and fluorescence self-quenching from dye-labeled gramicidin channels, we observed that the efficiency of spFRET and self-quenching is widely distributed, which reflects a broad distribution of conformations, including "silent" states of active channels. Our results strongly suggest a hitherto undetectable correlation between the multiple conformational states of the gramicidin channel and its closed and open states in a lipid bilayer. We have recently applied this new single-molecule experimental approach for studying ion-channel conformational dynamics and mechanisms of NMDA receptors in living cells.
As a crucial and early event in the DNA repair process, DNA damage recognition involves complex protein-DNA and protein-protein interactions. Our single-molecule spectroscopy study reveals dynamics of fluctuating molecular non-covalent interactions within single XPA-DNA complexes that are involved in the DNA (NER) damage recognition processes. These results have facilitated a molecule-level understanding of the dynamics and mechanisms of the damage-recognition process in DNA repair. We observed fluorescence-intensity fluctuations in single XPA-DNA complexes at two different time-scales. We concluded that the fast conformational change coordinates are associated with DNA structural fluctuations, but slow conformational coordinates are attributed to interactive motions of the XPA-DNA complex at the binding site, a finding consistent with XPA’s ability to recognize a wide variety of DNA lesions. The required, slow and large-amplitude conformational motions of XPA-DNA complexes have not been reported previously. Approximately 10-fold variation occurs in the rates of the slow interactive conformational motions among the individual complexes. This inhomogeneity is most likely associated with the existence of different subsets of protein conformations seeking an induced-fit to a conformationally fluctuating DNA lesion. Conformational dimensionality reduction, fly-casting driven by induced-fit, water and ion exclusion, DNA and peptide chain diffusive relaxation, protein interior packing relaxation, and hydrogen bond formations are all likely associated with the potential barriers for the trapped interactive states. These trapped and slow-fluctuating interactions could be crucial in XPA-DNA interaction dynamics and intrinsically pertinent to the protein-DNA complexes in other DNA damage recognition processes.
We have applied single-molecule spectroscopy and ultrafast spectroscopy on single molecule studies of photosensitized interfacial electron transfer (ET) processes in Coumarin 343 (C343)-TiO2 nanoparticle (NP) and Cresyl Violet (CV+)-TiO2 NP systems, using time-correlated single photon counting coupled with scanning confocal fluorescence microscopy. Fluorescence intensity trajectories of individual dye molecules adsorbed on a semiconductor NP surface showed fluorescence fluctuations and blinking, with time constants distributed from milliseconds to seconds. The fluorescence fluctuation dynamics were found to be inhomogeneous from molecule to molecule and from time to time, showing significant static and dynamic disorders in the interfacial ET reaction dynamics. We attribute fluorescence fluctuations to the interfacial ET reaction rate fluctuations, associating redox reactivity intermittency with the fluctuations of molecule-TiO2 electronic and Franck-Condon coupling. The inhomogeneous nanoscale molecule-surface and molecule-molecule interactions are presumably the origins of the complexity in interfacial ET dynamics. These nanoscale inhomogenities at interfaces or on surfaces make it highly difficult for ensemble-averaged measurements to dissect the complex interfacial ET processes. The difficulty comes from both spatial and temporal inhomogeneities, which can be identified, measured, and analyzed best by studying one molecule at a time in a specific nanoscale local environment. Intermittent interfacial ET dynamics of individual molecules could be characteristic of a surface chemical reaction strongly involved with and regulated by molecule-surface interactions. The intermittent interfacial reaction dynamics that likely occur among single molecules in other interfacial and surface chemical processes can typically be observed by single-molecule studies, but not by conventional ensemble-averaged experiments.
Single-molecule fluorescence intensity trajectories contain rich information about the molecular dynamics of protein conformational changes, DNA-protein interactions, and protein-protein interactions. However, the typical dynamic range of the single-molecule trajectories has only been from the sub-millisecond to second-and-longer. We have developed single-molecule spectroscopic capabilities to measure the single-molecule fluorescence lifetime trajectories, extending the time scale to the nanosecond regime. At the same time, we have initiated methods to obtain single molecule fluorescence anisotropy trajectories at the nanosecond-to-second time-scale and to achieve a nanoscale topographic characterization in correlations with single-molecule spectroscopy.
Single-Molecule Time-Resolved Fluorescence Anisotropy: Molecule rotational motion rates are dependent upon molecular hydrodynamic shapes and masses that can be changed by molecular interactions and complex formation/dissociation. Measuring the time-dependent anisotropy of a site-specifically labeled dye molecule would be a means of probing the conformational motions of the labeled domain of the protein. Because the time-scale for dye molecule spinning is in the sub-nanosecond range, the nanosecond-to-microsecond protein conformational motions can be differentiated and probed by measuring the time-dependent anisotropy of the dye molecules in this longer time regime. The results will be observed as changes in the fluorescence polarization anisotropy in time. This technique allows us to measure the single-molecule conformational changes as well as the substrate-enzyme complex formation, reaction, and dissociation in real time.
AFM-enhanced optical spectroscopic microscopy: We have been working to combine AFM and single-molecule microscopy in one instrument in order to obtain molecular scale topographic information and the correlated spectroscopic information for the same single-molecule reaction system. This approach enhances research in our protein conformational and enzymatic reaction dynamics project. Single-molecule dynamics have been "traditionally" studied using fluorescence spectroscopy that probes the overall molecular or active site conformational fluctuations and the excited-state lifetimes. Vibrational-mode resolved single-molecule dynamics has never been conducted due to the lack of vibrational specificity of fluorescence. Quite conceivably, Raman spectroscopy, including surface-enhanced Raman spectroscopy (SERS), could provide rich information on vibrational relaxation, vibrational coupling, chemical bond formation/dissociation, and mode-specific nuclear displacements. Based on the near-field enhancement at the metallic AFM tip, we have experimentally demonstrated AFM tip enhanced fluorescence lifetime imaging microscopy (AFM-FLIM) and currently working on demonstrating the AFM tip enhanced surface enhanced Raman spectroscopy (AFM-SERS) imaging guided by finite element method (FEM) computational simulation of the near-field electronmagnetic field distributions.
Approach
- Single-molecule fluorescence spectroscopy
- Nanoscale site-specific surface enhanced Raman spectroscopy
- Charge transfer enhanced Raman spectroscopy
- AFM enhanced single-molecule optical imaging and spectroscopy
- Near-field scanning optical microscopy
- Ultrafast spectroscopy and non-linear optical imaging
Updated: 09/12/2022 08:19AM